Atlantic Meridional Overturning Circulation
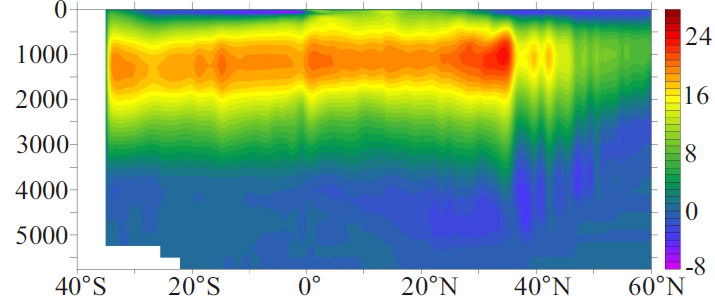
The Meridional Overturning Circulation in the Atlantic Ocean (AMOC) is an important component of the climate system. Knowledge of its stability and variability is essential to understand past and future climate fluctuations. My interest in the AMOC started with my PhD research at Utrecht University. Under mentorship of Will de Ruijter, I studied the stability of the AMOC with respect to Southern (specifically Agulhas Leakage) sources of heat and salt. We found that these sources could significantly impact the stability, as in particular the salt import could strengthen the AMOC and enhance its stability (Weijer et al. 1999; Weijer 2000; Weijer et al. 2001; Weijer et al. 2002).
I continued this work as a postdoc at the Institute for Marine and Atmospheric Research Utrecht (IMAU), working with Henk Dijkstra. We studied the thermohaline circulation in an idealized double hemispheric configuration, with equatorially symmetric surface forcing. In this context, equatorially asymmetric ("pole-to-pole") circulation patterns arise due to non-linear dynamics of the flow, despite symmetric forcing conditions. The question is whether the mechanism of symmetry breaking, as found in two-dimensional models, survives when three-dimensional effects (particularly rotation) are taken into account. An analysis of the energy transfer between the basic state and the most unstable perturbation showed that the mechanism underlying the symmetry breaking pitchfork bifurcation is essentially the same for two- and three-dimensional models of the thermohaline circulation (Weijer and Dijkstra 2001).
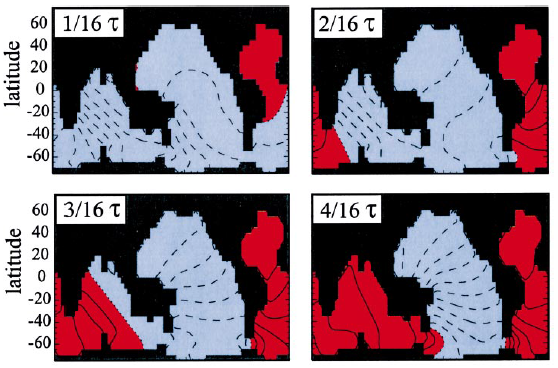
Working with Henk, I was involved in the development of a fully-implicit climate model, THCM (Weijer et al. 2003). This model uses concepts from dynamical systems theory and advanced numerical methods to solve steady patterns of the ocean circulation and their linear stability. For a 5 degree global configuration, we determined the least stable modes that threaten the stability of a decent global overturning circulation. Among the three least stable modes, two oscillatory modes were found, and one real mode. The oscillatory modes could be identified as overturning oscillations, for which temperature and salinity anomalies are advected by the global overturning circulation (Weijer and Dijkstra 2003).
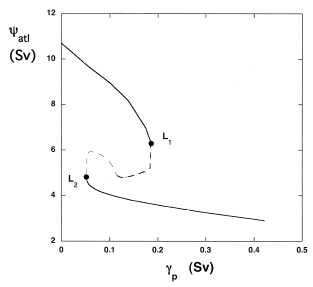
For an even higher resolution, we explicitly computed the classical hysteresis loop of the global overturning circulation with respect to North Atlantic freshwater input; not by time-integration and monitoring quasi-equilibrium behavior, but through parameter continuation. This allowed us to identify even the unstable branch connecting the conveyor belt circulation and the collapsed state (Dijkstra and Weijer 2003; Dijkstra and Weijer 2005).
Unfortunately, these implicit methods are limited to models with low resolution, which do not explicitly resolve eddies. In Weijer et al. (2012) we compared the sensitivity of the AMOC with respect to Greenland freshwater forcing in ocean models with different resolutions. We found that in a strongly-eddying ocean model the AMOC response is stronger than in a non-eddying, IPCC-class ocean model, and that the qualitative response is different as well. Explicitly resolving eddy transports is hence crucial for a correct representation of AMOC sensitivity.
This study was followed up by
den Toom et al. (2014),
where we addressed the question whether the salt advection feedback is
still active in a strongly eddying overturning circulation. No
evidence for this feedback was found, putting into question
whether concepts of AMOC stability learned from low-resolution
models carry over to the turbulent regime.
In 2019, this line of research culminated in a review and synthesis paper on the stability of the AMOC (Weijer et al. 2019). With this paper, we contributed to a special issue that marked the sunsetting of the US AMOC Science Team. I also contributed to a paper led by Joel Hirschi, addressing the representation of the AMOC in high-resolution models (Hirschi et al. 2020).
Given the importance of the AMOC for the global climate system and its response to external forcing, it is critical that climate models represent the AMOC correctly. In Weijer et al. (2020a) we evaluated the AMOC in 27 models that contributed simulations to the 6th phase of the Coupled Model Intercomparison Project (CMIP6). We found that the ensemble-mean AMOC is very close to observations, but significant model spread remains. We also found that most models project a stronger 21st century AMOC decline than in previous phase of CMIP (CMIP3, CMIP5). In fact, most models show the same AMOC trajectory at least until 2060, regardless of the scenario, suggesting that most of the AMOC has already been committed by historical emissions.
References
- Dijkstra and Weijer 2003: Stability of the global ocean circulation: the connection of equilibria in a hierarchy of models. J. Mar. Res., 61, 725-743, doi: 10.1357/002224003322981129.
- Dijkstra and Weijer 2005: Stability of the global ocean circulation: basic bifurcation diagrams. J. Phys. Oceanogr., 35, 933-948, doi: 10.1175/jpo2726.1.
- Hirschi et al. 2020: The Atlantic meridional overturning circulation in high resolution models. J. Geophys. Res., 125, e2019JC015522, doi: 10.1029/2019JC015522.
- den Toom et al. 2014: Sensitivity of a strongly eddying global ocean to North Atlantic freshwater perturbations. J. Phys. Oceanogr., 44, 464-481, doi: 10.1175/jpo-d-12-0155.1.
- Weijer and Dijkstra 2001: A bifurcation study of the three-dimensional thermohaline ocean circulation: the double-hemispheric case. J. Mar. Res., 59, 599-631, doi: 10.1357/002224001762842208.
- Weijer and Dijkstra 2003: Multiple oscillatory modes of the global ocean circulation. J. Phys. Oceanogr., 33, 2197-2213, doi: 10.1175/1520-0485(2003)033<2197:momotg>2.0.co;2.
- Weijer et al. 1999: Impact of interbasin exchange on the Atlantic overturning circulation. J. Phys. Oceanogr., 29, 2266-2284, doi: 10.1175/1520-0485(1999)029<2266:ioieot>2.0.co;2.
- Weijer et al. 2001: Stability of the Atlantic overturning circulation: Competition between Bering Strait freshwater flux and Agulhas heat and salt sources. J. Phys. Oceanogr., 31, 2385-2402, doi: 10.1175/1520-0485(2001)031<2385:sotaoc>2.0.co;2.
- Weijer et al. 2002: Response of the Atlantic overturning circulation to South Atlantic sources of buoyancy. Glob. Planet. Change, 34, 293-311, doi: 10.1016/s0921-8181(02)00121-2.
- Weijer et al. 2003: A fully-implicit model of the global ocean circulation. J. Comp. Phys., 192, 452-470, doi: 10.1016/j.jcp.2003.07.017.
- Weijer et al. 2012: Response of the Atlantic Ocean circulation to Greenland ice sheet melting in a strongly-eddying ocean model. Geophys. Res. Lett., 39, L09606, doi: 10.1029/2012GL051611.
- Weijer et al. 2019: Stability of the Atlantic Meridional Overturning Circulation: A review and synthesis. J. Geophys. Res., 124, 5336-5375, doi: 10.1029/2019JC015083.
- Weijer et al. 2020. CMIP6 Models Predict Significant 21st Century Decline of the Atlantic Meridional Overturning Circulation. Geophys. Res. Lett., 47, e2019GL086075, doi: 10.1029/2019GL086075.